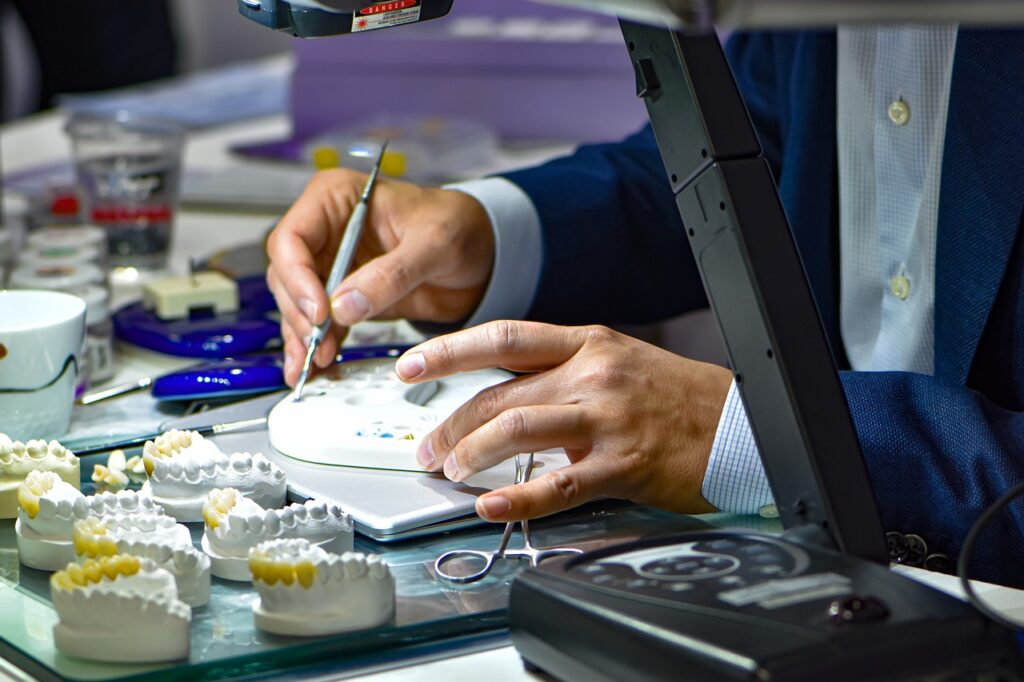
Implant Materials and Surface Modifications: Enhancing Osseointegration and Long-term Stability
- Author:gulrukhsalman@gmail.com
- July 16, 2024
- No Comments
Dental implants have revolutionized the field of restorative dentistry, providing a reliable and durable solution for tooth replacement. Central to the success of dental implants is the phenomenon of osseointegration, which refers to the direct structural and functional connection between living bone and the surface of a load-bearing artificial implant. The development of new implant materials and surface modifications is critical to enhancing osseointegration and improving long-term implant stability. This article explores the current state of implant materials, the latest surface modification techniques, research advances, and future directions in this dynamic field.
Current Implant Materials
The most commonly used materials for dental implants are titanium and its alloys, primarily due to their excellent biocompatibility, mechanical properties, and ability to form a stable oxide layer that enhances osseointegration. Titanium implants have been the gold standard for decades, providing predictable outcomes and high success rates. However, the quest for improved performance and reduced healing times has driven the exploration of alternative materials.
Zirconia, a ceramic material, has gained attention as a potential alternative to titanium. Zirconia implants offer several advantages, including high biocompatibility, excellent aesthetic properties due to their tooth-like color, and resistance to corrosion and plaque accumulation. Clinical studies have shown promising results with zirconia implants, indicating comparable success rates to titanium implants. However, concerns regarding their mechanical properties, such as brittleness and lower fracture toughness compared to titanium, have limited their widespread adoption. Ongoing research aims to address these limitations through material enhancements and innovative manufacturing techniques.
Surface Modifications for Enhanced Osseointegration
The surface characteristics of dental implants play a crucial role in osseointegration. Various surface modification techniques have been developed to enhance the bone-implant interface and improve long-term stability. These techniques can be broadly categorized into physical, chemical, and biochemical modifications.
Physical modifications involve altering the topography of the implant surface to increase its roughness and surface area. Methods such as grit blasting, acid etching, and plasma spraying create micro- and nano-scale roughness that promotes cellular adhesion and proliferation. Studies have demonstrated that rougher surfaces enhance the initial mechanical interlocking between the implant and bone, leading to improved osseointegration. Additionally, the combination of different physical modification techniques has shown synergistic effects, further enhancing the surface properties of implants.
Chemical modifications involve altering the surface chemistry of the implant to enhance its bioactivity. Techniques such as anodization, alkali treatment, and hydrothermal treatment create a bioactive surface that can promote the deposition of bone-like apatite. These chemically modified surfaces enhance the wettability and protein adsorption, facilitating the attachment and differentiation of osteogenic cells. Furthermore, the incorporation of bioactive elements such as calcium, phosphorus, and magnesium into the implant surface has shown promising results in enhancing osseointegration.
Biochemical modifications involve coating the implant surface with bioactive molecules, growth factors, or peptides that promote bone formation and integration. One of the most widely studied approaches is the immobilization of bone morphogenetic proteins (BMPs) onto the implant surface. BMPs are potent osteoinductive factors that stimulate the recruitment and differentiation of osteoprogenitor cells. Preclinical and clinical studies have demonstrated that BMP-coated implants significantly enhance osseointegration and bone regeneration. However, challenges related to the controlled release and long-term stability of these bioactive molecules need to be addressed for their successful clinical application.
Research Advances
Recent advances in nanotechnology have opened new avenues for implant surface modifications. Nanostructured surfaces, characterized by features on the nanometer scale, mimic the natural extracellular matrix and provide a favorable microenvironment for cellular interactions. Nanotubes, nanowires, and nanoporous surfaces have been developed using techniques such as electrochemical anodization and chemical vapor deposition. These nanostructured surfaces enhance protein adsorption, cell adhesion, and osteogenic differentiation, leading to improved osseointegration. Additionally, the incorporation of nanoparticles into the implant surface has shown potential for delivering therapeutic agents and growth factors in a controlled manner, further enhancing the regenerative potential of implants.
Another promising area of research is the development of bioactive coatings that promote specific cellular responses. Bioactive glass, a type of biocompatible glass with osteoinductive properties, has been extensively studied for coating dental implants. Bioactive glass coatings form a strong bond with bone tissue and stimulate the release of ions that promote osteogenesis. Clinical studies have shown improved osseointegration and bone regeneration with bioactive glass-coated implants. Furthermore, composite coatings combining bioactive glass with polymers or other ceramics have shown synergistic effects, enhancing the mechanical properties and bioactivity of the implant surface.
The use of growth factors and signaling molecules for surface modification is another exciting research direction. In addition to BMPs, other growth factors such as vascular endothelial growth factor (VEGF) and platelet-derived growth factor (PDGF) have shown potential in enhancing angiogenesis and osteogenesis. Combining multiple growth factors in a controlled release system can create a synergistic effect, promoting both vascularization and bone formation. However, challenges related to the delivery, stability, and cost of these growth factors need to be addressed before their widespread clinical use.
Critical Analysis
While significant progress has been made in the development of implant materials and surface modifications, several challenges and limitations remain. Titanium and its alloys, despite their excellent properties, are still prone to issues such as peri-implantitis and allergic reactions in some patients. The development of alternative materials like zirconia offers potential solutions, but their mechanical properties need further optimization to ensure long-term durability.
Surface modification techniques have shown promising results in enhancing osseointegration, but the variability in clinical outcomes and lack of standardized protocols pose challenges. The long-term stability and biological response of modified surfaces need to be thoroughly investigated through rigorous preclinical and clinical studies. Additionally, the cost and complexity of advanced surface modification techniques may limit their accessibility and widespread adoption in clinical practice.
The integration of nanotechnology and bioactive coatings has shown great potential, but challenges related to scalability, reproducibility, and regulatory approval need to be addressed. The controlled release of bioactive molecules and growth factors remains a significant challenge, requiring innovative delivery systems that can provide sustained and localized release over extended periods. Furthermore, the potential risks and side effects of these advanced materials and coatings need to be carefully evaluated to ensure patient safety.
Future Directions
The future of implant materials and surface modifications lies in the development of smart and multifunctional implants. Smart implants
, equipped with sensors and responsive coatings, have the potential to monitor the local biological environment and release therapeutic agents in response to specific stimuli. For instance, implants could be designed to detect early signs of inflammation or infection and respond by releasing anti-inflammatory or antimicrobial agents, thus preventing complications and promoting healing. Research into such responsive systems is still in its early stages, but the integration of advanced materials science and biomedical engineering holds great promise.
Bioprinting is another exciting avenue for the future of dental implants. Using 3D bioprinting technology, patient-specific implants can be created with precise anatomical accuracy and tailored surface properties. This technology allows for the incorporation of multiple materials and bioactive agents in a single construct, facilitating the regeneration of complex tissue structures. Bioprinting also enables the creation of porous implants that closely mimic the natural bone architecture, enhancing vascularization and integration with the host tissue.
Gene therapy represents a revolutionary approach to enhancing osseointegration. By delivering specific genes that encode osteogenic factors directly to the implant site, it is possible to stimulate the local production of these factors, promoting bone formation and integration. This technique could overcome the limitations of exogenous growth factor delivery by ensuring a sustained and localized production of osteogenic proteins. However, challenges related to gene delivery vectors, control of gene expression, and potential off-target effects need to be carefully addressed.
Artificial intelligence (AI) and machine learning (ML) are poised to play a transformative role in implantology. AI and ML can be used to analyze vast amounts of clinical data to identify patterns and predict outcomes, leading to more personalized and effective treatment plans. These technologies can also optimize the design and manufacturing processes of implants, ensuring better fit and function. Furthermore, AI-driven diagnostic tools can aid in the early detection and management of peri-implant diseases, improving long-term outcomes.
Collaborative research efforts between materials scientists, bioengineers, and clinicians will be crucial in advancing the field of implant materials and surface modifications. Interdisciplinary approaches will enable the development of innovative solutions that address the complex challenges associated with osseointegration and implant stability. Additionally, regulatory frameworks need to evolve to accommodate the rapid advancements in technology and ensure the safety and efficacy of new materials and techniques.
Educational initiatives aimed at training clinicians in the latest advancements and best practices in implantology are essential for the successful adoption of new technologies. Continuous professional development programs, workshops, and conferences provide platforms for knowledge exchange and skill enhancement, ensuring that dental professionals are well-equipped to deliver optimal care to their patients.
In conclusion, the development of new implant materials and surface modifications is a dynamic and rapidly evolving field. Titanium and zirconia remain the primary materials used for dental implants, each with its advantages and limitations. Surface modification techniques, including physical, chemical, and biochemical methods, have significantly enhanced osseointegration and implant stability. Recent advances in nanotechnology, bioactive coatings, and growth factor delivery have further expanded the possibilities for improving implant performance. However, challenges related to material properties, clinical variability, cost, and regulatory approval need to be addressed. The future of implant materials and surface modifications lies in the integration of smart technologies, bioprinting, gene therapy, and AI, promising to revolutionize dental implantology and improve patient outcomes. Continued research, interdisciplinary collaboration, and education will be key to unlocking the full potential of these advancements and ensuring their successful translation into clinical practice.